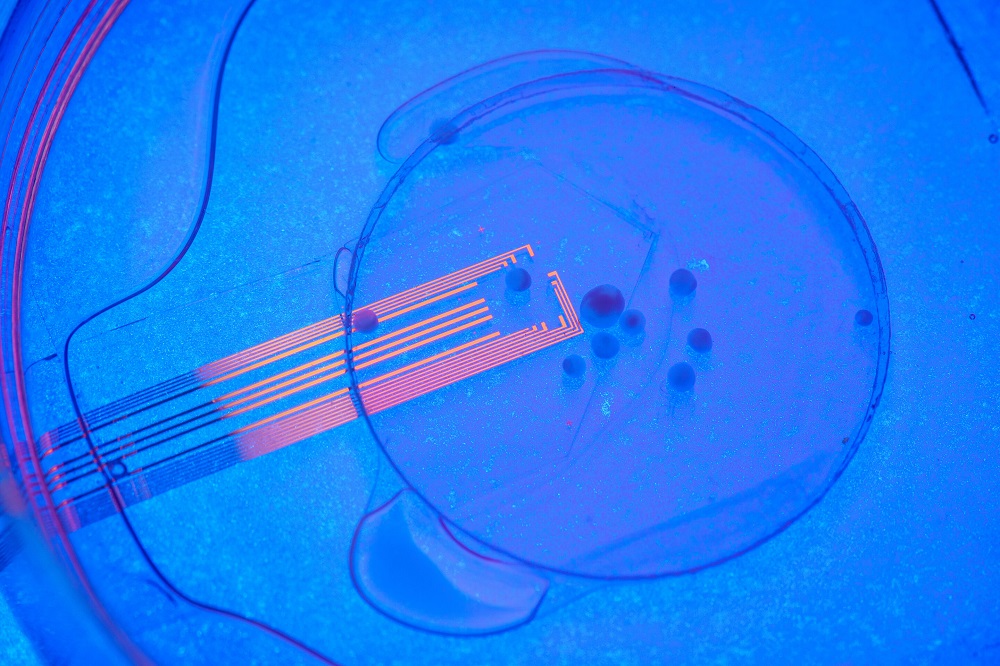
Could these mini-brain ‘grafts’ be used to repair brain injuries in the future?
In a world’s first, researchers have shown that human brain organoids transplanted into live animals’ brains successfully formed human-mouse synaptic connections and reacted to visual stimuli. The results, made possible by an unprecedented multi-faceted technology, show the cross-species transplant merged with the animal’s visual cortex and tapped into the local blood supply.
The study from UC San Diego revolves around brain organoids or mini-brains – structures designed to resemble a specific brain region or to simulate isolated changes caused by a neurological disorder.
To date, scientists have successfully implanted human brain organoids into animal tissue, hoping these structures can repair or replace damaged tissue in the brain to act as a neural prosthesis. And although these studies hold promise for neuroscientific research, scientists have still been unable to prove that human brain organoids implanted in the animal brain could merge with their native structures and react to stimuli along with them–Until now.
In the new study, the UC San Diego researchers developed a new type of ultra-sensitive electrode to record neuronal activity and combined it with a technology called two-photon imaging, which captures live cell activity in biological tissue.
Using the electrodes, they recorded neural activity from the implanted organoid, showing that it had formed functional connections with the mouse cortex and reacted to external stimuli. Through the two-photon imaging, they were able to observe that mouse blood vessels grew into the organoid, providing it with nutrients and oxygen– a big deal as organoids do not contain vascular cells.
“No other study has been able to record optically and electrically at the same time,” states Madison Wilson, the paper’s first author and Ph.D. candidate in Professor Duygum Kuzum’s group at UC San Diego. “Our experiments reveal that visual stimuli evoke electrophysiological responses in the organoids, matching the responses from the surrounding cortex.”
Unprecedented imaging
The organoids used in this study were human cortical organoids designed to typify some, but not all, of the features of the cerebral cortex. The team grew the mini-brains from differentiated human induced pluripotent stem cells: special cells created from adult cells (in this case, skin cells) exposed to a chemical cocktail to revert them back to their embryonic state.
To measure the activity of the organoid once transplanted into the mouse cortex, the team used platinum nanoparticles to lower a special property of graphene electrodes known as impedance – which blocks or ‘resists’ the flow of electricity. Once they had achieved this, 100 times more of the electrical potential created by neurons could flow through the electrodes, enabling this activity to be recorded and analyzed.
Amazingly, the scientists achieved this while keeping the electrodes transparent to lower the electrical resistance and increase conductivity while viewing the activity of neurons directly beneath the arrays.
Duygu Kuzum, a professor of electrical and computer engineering at UC San Diego, says, “By lowering impedance, we can shrink electrode dimensions down to single cell size and record neural activity with single cell resolution.”
In tests on mice, these highly sensitive graphene electrodes could record and image neuronal activity at both the macroscale and single-cell levels. Accordingly, the data indicated that the neurons within the organoids became synchronized with the surrounding tissue. At the same time, the two-photon imaging clearly showed the organoid’s integration with the mouse’s cortex and blood supply to back these results.
Live brain implants
Taken together, the team states their multi-modal imaging platform indicates that human and mouse cortical tissues established functional connections enabling the organoid to react to external sensory stimuli.
They later verified their findings during dissection, where the organoid was stained with two different types of antibodies – one that ‘traces’ human cells and the other that marks synaptic connections. Via this investigation, the team confirmed that the human cells had traveled as far as 4mm away from the boundary of the graft site migrating along the mouse’s corpus callosum, a thick nerve tract just below the cerebral cortex – providing further proof of integration.
In their white paper, the team writes this combination of stem cell and neurorecording technologies could herald the next generation of disease models, drug screening and personalized medicine platforms, and transplantable neural prosthetics to restore specific lost, degenerated, or damaged brain regions.
“We envision that, further along the road, this combination of stem cells and neurorecording technologies will be used for modeling disease under physiological conditions; examining candidate treatments on patient-specific organoids; and evaluating organoids’ potential to restore specific lost, degenerated or damaged brain regions,” Kuzum concludes.